Chemical Kinetics
What is Chemical Kinetics:
Chemical kinetics, also called reaction kinetics, helps us understand the rates of reactions and how it is influenced by certain conditions. It further helps to gather and analyze information about the mechanism of the reaction and define the characteristics of a chemical reaction.
Rate of Formation and Rate of Disappearance:
In any chemical reaction, as the reaction proceeds, the amount of reactants decreases, whereas the amount of products increases. One has to understand that the rate of the overall reaction depends on the rate at which reactants are consumed or the rate at which the products are formed.
If a graph is plotted between the concentration of reactants and products and time, the rate of formation of products and the rate of disappearance of reactants can be easily calculated from the slope of curves for products and reactants. The overall rate of the reaction may or may not be equal to the rate of formation and rate of disappearance.
(a) Product concentration is zero at time t = 0
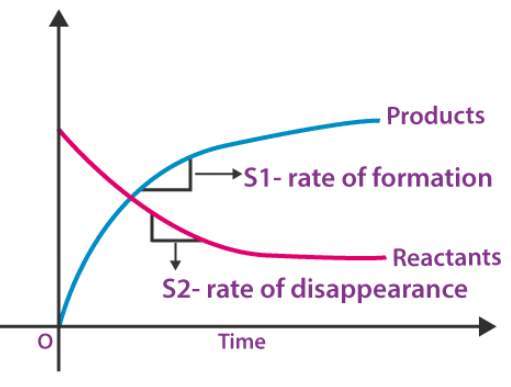
(b) At time t = 0, both reactants and products are present.
From the graph, it is understood that the slope of the reactants curve is negative and that for product curve is positive, indicating the concentration of reactants and products decreases and increases, respectively. We will take a simple reaction as an example to illustrate how the rate of overall reactions, rate of disappearances of reactants and rate of formation of products are related.
Let us take the reaction of the formation of water.
2H2 + O2 → 2H2O
From the balanced equation, it is under that for one mole of O2 consumed, 2 moles of H2 will be consumed, and 2 moles of H2O will be formed. Say, the reaction proceeds for 10 mins, taking 1 mole of H2 and O2 each in the reaction mixture.
2H2 + O2 → 2H2O
t = 10 mins 1 – 0.5 1 – 0.25 0.5
Say after 10 minutes, 0.5 moles of H2 is consumed, and according to stoichiometry, 0.25 moles of O2 is consumed, and 0.5 moles of H2O is formed. Now, let us calculate the rates for H2, O2 and H2O for the first 10 minutes.
From the above calculations, we can see that rate at which H2 is consumed is twice the rate at which O2 is consumed. So, the stoichiometry of the reaction relates rates of formation and disappearances of different reactants and products as follows.
Let, aA + bB → cC + dD, be a reaction.
Average and Instantaneous Rate:
The rate of reaction can be classified into average and instantaneous rates depending on the amount of time period. If the time period taken is finite, then it’s called the average rate and is represented as,
ravg = Δ[C]/ Δt
Δ[C] is the change in concentration
Δt is the change in time
ravg is the average rate
The average rate doesn’t give exact information in most cases about the completion of the reaction.
Example:
Let us consider the hydrolysis of esters to form acid and alcohol.
Say, at time t = 0, and there was 1 M solution of ester which becomes 0.5 M in 30 mins. So, it is logical for us to assume that the reaction will be 100% completed in 1 hour. But in reality, the reaction takes more than 3 hours to reach completion. So, to get a broader insight into the time taken for completion and other purposes, Instantaneous rate is used, which is represented as:
From above, it’s understood that the time period taken is almost zero, from t = 0 to t = 0.0000…1 second. This eventually comes out to be the differential of change in concentration with respect to time.
For all practical purposes, the instantaneous rate is used, which can be calculated from the concentration in the time graph by finding a tangent at a point.
The unit of rate is Mol L-1 s-1 because it is concentration/time, and concentration is expressed in terms of Molarity/mol l-1). It can also be Nm-2/s if the active mass is used in terms of partial pressures. Depending on other units of time, it can also be mol l-1 min-1 or mol l-1 hour-1 etc.
Factors Affecting the Reaction Rate:
The rate of a reaction can be altered if any of the following parameters are changed:
Concentration of Reactants:
According to collision theory, reactant molecules collide with each other to form products. If the concentration of reactants is increased, the number of colliding particles will increase, thereby increasing the rate of reaction.
Nature of the Reactants:
The reaction rate also depends on the types of substances that are reacting. If we consider acid/base reactions, salt formation and ion exchange, they are mostly fast reactions. During the formation of a covalent bond between the molecules that results in the formation of larger molecules, the reaction that takes place is usually slower. Furthermore, the nature and strength of bonds in reactant molecules significantly affect the rate of their transformation into products.
Physical State of Reactants:
The physical state of a reactant, whether it is solid, liquid or gas, can greatly affect the rate of change. To discuss it further, if reactants are in the same phase, let’s say they are in an aqueous solution, here the thermal motion will bring them together. If they are in different phases, then the reaction will be limited to the interface between the reactants. The reaction mainly occurs only at their area of contact, in the case of a liquid and a gas, at the surface of the liquid.
Surface Area of Reactants:
If we take two solids, the particles that are at the surface will take part in the reaction. Likewise, if we want to crush a solid into smaller parts, more particles will be present at the surface. What it means is that the frequency of collisions between these and reactant particles will most likely increase. As a result, the reaction will occur more rapidly.
When two or more reactants are in the same phase of fluid, their particles collide more often than when either or both are in the solid phase or when they are in a heterogeneous mixture. In a heterogeneous medium, the collision between the particles occurs at an interface between phases. Compared to the homogeneous case, the number of collisions between reactants per unit time is significantly reduced, and so is the reaction rate.
Temperature:
If the temperature is increased, the number of collisions between reactant molecules per second (frequency of collision) increases, thereby increasing the rate of the reaction. But depending on whether the reaction is endothermic or exothermic, an increase in temperature increases the rate of forward or backward reactions, respectively.
In a system where more than one reaction is possible, the same reactants can produce different products under different temperature conditions.
At 100 0C in the presence of dilute sulphuric acid, diethyl ether is formed from ethanol.
2CH3CH2OH → CH3CH2OCH2CH3+H2O
At 180 0C in the presence of dilute sulphuric acid, ethylene is the major product.
CH3CH2OH → C2H4+H2O
Effect of Solvent:
The nature of the solvent also depends on the reaction rate of the solute particles.
Example:
When sodium acetates react with methyl iodide, it gives methyl acetate and sodium iodide.
CH3CO2Na(sol)+CH3I(liq)→CH3CO2CH3(sol)+NaI(sol)
The above reaction occurs faster in organic solvents such as DMF (dimethylformamide) than in CH3OH (methanol) because methanol is able to form a hydrogen bond with CH3CO2 – but not with DMF.
Reaction Order:
The order of reaction can be defined as the power dependence of rate on the concentration of all reactants. For example, the rate of a first-order reaction is dependent solely on the concentration of one species in the reaction. Some characteristics of the reaction order for a chemical reaction are listed below:
- Reaction order represents the number of species whose concentration directly affects the rate of reaction.
- It can be obtained by adding all the exponents of the concentration terms in the rate expression.
- The order of reaction does not depend on the stoichiometric coefficients corresponding to each species in the balanced reaction.
- The reaction order of a chemical reaction is always defined with the help of reactant concentrations and not with product concentrations.
- The value of the order of reaction can be in the form of an integer or a fraction. It can even have a value of zero.
In order to determine the reaction order, the power-law form of the rate equation is generally used. The expression of this form of the rate law is given by:
r = k[A]x[B]y.
In the expression described above, ‘r’ refers to the rate of reaction, ‘k’ is the rate constant of the reaction, [A] and [B] are the concentrations of the reactants. The exponents of the reactant concentrations x and y are referred to as partial orders of the reaction. Therefore, the sum of all the partial orders of the reaction yields the overall order of the reaction.
How to Determine Reaction Order?:
There are several different methods which can be followed in order to determine the reaction order. Some of these methods are described in this subsection.
Initial Rates Method:
- First, the natural logarithm form of the power-law expression is obtained. It is given by: ln(r) = ln(k) + x.ln([A]) + y.ln([B]) + ….
- The partial order corresponding to each reactant is now calculated by conducting the reaction with varying concentrations of the reactant in question and the concentration of the other reactants kept constant.
- If the partial order of A is being determined, the power-law expression of the rate equation now becomes ln(r) = x.ln([A]) + C, where C is a constant.
- A graph is now plotted by taking ‘ln(r)’ as a function of ln([A]), the corresponding slope is the partial order, given by x.
Integral Method:
- The order of reaction obtained from the initial rates method is usually verified using this method.
- The measured concentrations of the reactants are compared with the integral form of the rate law.
- For example, the rate law for a first-order reaction is verified if the value for ln[A] corresponds to a linear function of time; integrated rate equation of a first-order reaction:
ln([A]) = -kt + ln([A]0).
Differential Method:
- This method is the easiest way to obtain the order of reaction
- First, the rate expression of the reaction is written (r = k[A]x[B]y..)
- The sum of the exponents x+y+… gives the final value of the reaction order.
Apart from these methods, there exist other ways to obtain the reaction order, such as the method of flooding in which the concentration of a single reactant is measured when all the other reactants are present in huge excess.
Different Values of Reaction Order:
As discussed earlier, the value of the order of reaction may be in the form of an integer, zero, or a fraction. A graph detailing the reaction rates for different reaction orders can be found below.
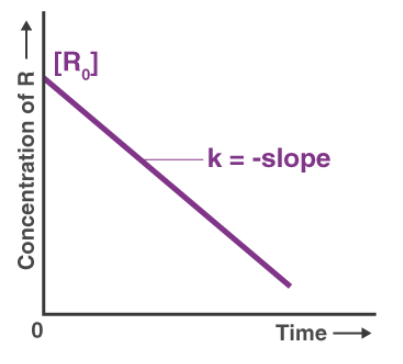
Chemical reactions can be classified into the following types based on the dependence of the rate on the concentration.
Zero Order Reactions:
- The rate of reaction is independent of the concentration of the reactants in these reactions.
- A change in the concentration of the reactants has no effect on the speed of the reaction
- Examples of these types of reactions include the enzyme-catalyzed oxidation of CH3CH2OH (ethanol) to CH3CHO (acetaldehyde).
First-Order Reactions:
- The rates of these reactions depend on the concentration of only one reactant, i.e. the order of reaction is 1.
- In these reactions, there may be multiple reactants present, but only one reactant will be of first-order concentration while the rest of the reactants would be of zero-order concentration.
- Example of a first-order reaction: 2H2O2 → 2H2O + O2
Pseudo-First Order Reactions:
- In a pseudo-first order reaction, the concentration of one reactant remains constant and is therefore included in the rate constant in the rate expression.
- The concentration of the reactant may be constant because it is present in excess when compared to the concentration of other reactants, or because it is a catalyst.
- Example of a pseudo-first order reaction: CH3COOCH3 + H2O → CH3COOH + CH3OH (this reaction follows pseudo-first order kinetics because water is present in excess).
Second-Order Reaction:
- When the order of a reaction is 2, the reaction is said to be a second-order reaction.
- The rate of these reactions can be obtained either from the concentration of one reactant squared or from the concentration of two separate reactants.
- The rate equation can correspond to r = k[A]2 or r = k[A][B]
- Example of a second-order reaction: NO2 + CO → NO + CO2
Difference Between Molecularity and Order of Reaction
The molecularity of a reaction refers to the number of atoms, molecules, or ions which must undergo a collision with each other in a short time interval for the chemical reaction to proceed. The key differences between molecularity and reaction order are tabulated below.
Molecularity | Order of Reaction |
It is always a whole number | It can be a whole number or a fraction. |
It can be determined from the balanced chemical equation | It must be determined experimentally |
Is only applicable in simple reactions | The reaction order is applicable in all chemical reactions |
It can be noted that when the order of reaction is a fraction, the reaction is generally a chain reaction or follows some other complex mechanism. An example of a chemical reaction with a fractional reaction order is the pyrolysis of acetaldehyde. This reaction has an order of 1.5.
What is Reaction Half-Life?:
The half-life of a chemical reaction can be defined as the time taken for the concentration of a given reactant to reach 50% of its initial concentration (i.e. the time taken for the reactant concentration to reach half of its initial value). It is denoted by the symbol ‘t1/2’ and is usually expressed in seconds.
Half-Life Formula:
It is important to note that the formula for the half-life of a reaction varies with the order of the reaction:
- For a zero-order reaction, the mathematical expression that can be employed to determine the half-life is: t1/2 = [R]0/2k
- For a first-order reaction, the half-life is given by: t1/2 = 0.693/k
- For a second-order reaction, the formula for the half-life of the reaction is: 1/k[R]0,
where,
- t1/2is the half-life of the reaction (unit: seconds)
- [R0] is the initial reactant concentration (unit: mol.L-1 or M)
- k is the rate constant of the reaction (unit: M(1-n)s-1 where ‘n’ is the reaction order)
Derivation of Half-Life Formula for Zero-Order Reactions:
For a zero-order reaction, the units of the rate constant are mol.L-1.s-1. The expression for a zero-order rate constant is:
Substituting t = t1/2, at which point [R] = [R]0/2 (at the half-life of a reaction, reactant concentration is half of the initial concentration).
Rearranging the equation, the expression for the half-life of a zero-order reaction is found to be:
Derivation of Half-Life Formula for First-Order Reactions:
For a first-order reaction, the rate constant can be mathematically expressed as follows:
From the definition of reaction half-life, at t = t1/2, [R] = [R]0/2. Substituting these values in the expression for the first-order rate constant, the following equation is obtained:
Rearranging the expression to find the value of t1/2:
Thus, the half-life of a first-order reaction is given by 0.693/k.
Derivation of Half-Life Formula for Second-Order Reactions:
The half-life of a chemical reaction is the time taken for half of the initial amount of reactant to undergo the reaction.
Therefore, while attempting to calculate the half-life of a reaction, the following substitutions must be made:
[R] = [R]0/2,
and,
t = t1/2
Now, substituting these values in the integral form of the rate equation of second order reactions, we get:
Therefore, the required equation for the half life of second order reactions can be written as follows.
This equation for the half life implies that the half life is inversely proportional to the concentration of the reactants.
For more insight on these topics, please visit this website: CHEMICAL KINETICS